Geneious Keygen Jared
Features c file c31954282fcc7 Geneious R7 7.1.5.rar 1. Click on the download links. Wait for 5 seconds and then click on. 0 Comments.hencet abg pro tools 7 serial, Keygen Typing Assistant English 5 4 key para 5 2 08 Crack kk Bandicam 1 7 2 138 crack screenflow 2 1 4 mac Siemens Football Manager.
Abstract Premise of the study: Single-nucleotide polymorphism (SNP) marker discovery in plants with complex allotetraploid genomes is often confounded by the presence of homeologous loci (along with paralogous and orthologous loci). Here we present a strategy to filter for SNPs representing orthologous loci.
Methods and Results: Using Illumina next-generation sequencing, 54 million reads were collected from restriction enzyme–digested DNA libraries of a diversity of Gossypium taxa. Loci with one to three SNPs were discovered using the Stacks software package, yielding 25,529 new cotton SNP combinations, including those that are polymorphic at both interspecific and intraspecific levels. Frequencies of predicted dual-homozygous (aa/bb) marker polymorphisms ranged from 6.7–11.6% of total shared fragments in intraspecific comparisons and from 15.0–16.4% in interspecific comparisons. Conclusions: This resource provides dual-homozygous (aa/bb) marker polymorphisms. Both in silico and experimental validation efforts demonstrated that these markers are enriched for single orthologous loci that are homozygous for alternative alleles. Cottons ( Gossypium L. Spp.) provide the leading natural fiber for textiles, as well as an important seed product for feed, food, and oil.
The most widely grown species are the allotetraploids G. (upland cotton) and G. Barbadense L. (Sea Island cotton).
These species are both descended from an allopolyploidization event involving an A-genome diploid species, related to modern G. Arboreum L., and D-genome diploid species, related to modern G. Raimondii Ulbr. Recent developments in next-generation sequencing (NGS) technology have lowered the cost of sequencing per base, and enabled the genotyping by sequencing (GBS) approach for developing informative single-nucleotide polymorphism (SNP) markers in species with large, complex genomes , including species without a reference genome sequence. In this study, we employed a simple and cost-effective GBS approach to identify intraspecific and interspecific SNPs within and between allotetraploid cottons G. Hirsutum and G.
A major difficulty in the characterization and utilization of SNPs in polyploid species is determining whether a polymorphism detected by short-read NGS is the result of alternative alleles at a single locus or the presence of multiple homeologous loci. To identify markers that were likely to represent alternative alleles at a single orthologous locus, we used the Stacks bioinformatics pipeline as a filter to enrich for codominant markers composed of pairs of alleles that were homozygous in the respective taxa used for comparison. Given high enough sequence coverage to accurately identify all relevant alleles and loci, the sstacks algorithm implemented by Stacks assigns an aa/bb marker type in this situation (Scenario 1). In contrast, detection of polymorphisms between homeologous loci will likely give rise to an ab/ab marker type prediction (Scenario 2). Detection of a polymorphism between paralogs within a subgenome will give rise to an aa/ab marker type (Scenario 3). Markers that are homozygous in one parent and heterozygous in the other parent will also give rise to an aa/ab marker type prediction (Scenario 4). A host of other combinations will give rise to either ab/ab or aa/bb patterns (not shown).
Predicted marker type categories from the sstacks algorithm for four common genetic scenarios (out of many) that give rise to apparent GBS polymorphisms between two allotetraploids. Red lines indicate sequences that can be clearly assigned to the A T subgenome, and blue lines indicate those that can be assigned to the D T subgenome. Gray lines indicate regions of high sequence similarity between homeologs or paralogs (e.g., no differences outside of the SNP of interest).
Marker type predictions are based on the assumption that there is adequate sequence coverage to accurately score all alleles at all relevant loci. Given the array of possible scenarios that can give rise to candidate SNPs using GBS, we focused our efforts on markers with a simple aa/bb biallelic pattern. We considered these to be the best candidates for single loci with codominant polymorphisms between cotton accessions that would be useful for downstream applications such as genetic diversity studies, linkage and QTL mapping, genome-wide association studies (GWAS), and marker-assisted selection. METHODS AND RESULTS GBS was performed by a method similar to the general strategy outlined previously , with major differences. Genomic DNAs from cotton taxa were extracted from liquid N 2 flash-frozen seedlings using a protocol described previously with the addition of 1/10th volume of Plant RNA Isolation Aid (Ambion, Austin, Texas, USA) to the initial extraction buffer.
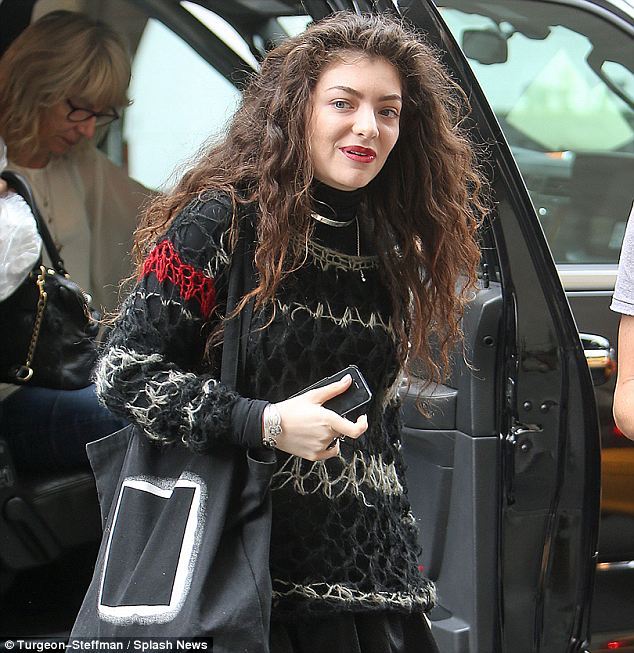
Genomic DNA (250 ng) was digested with 10 units of restriction endonucleases HinP1I or BsrGI for 2 h at 37°C. HinP1I is a CpG methylation-sensitive four-base cutter (G CGC), while BsrGI is a methylation-insensitive six-base cutter (T GTACA). Adapter ligations were carried out in 50-μL reactions in the presence of 40 pmol of the restriction-enzyme appropriate combination of annealed, 6-bp bar-coded, Illumina-compatible P5 and P7 adapters and 1600 cohesive-end ligation units of T4 DNA ligase (New England Biolabs, Ipswich, Massachusetts, USA) for 1 h at 37°C. Because active restriction enzymes were still present in these reactions, a final incubation for 30 min at 37°C was performed to cleave any chimeric ligation products between genomic DNA fragments (the adapters were designed to abolish the HinP1I or BsrGI recognition site upon ligation). All samples were pooled and then purified using MinElute columns (QIAGEN, Valencia, California, USA). Single-copy regions of the plastid genome can be present in 1500 copies per cell in leaf tissue and may thus represent a major contaminant of GBS libraries.
In silico restriction digestion of the G. Hirsutum plastid genome showed that no fragments were present between 186 bp and 218 bp in HinP1I digests and between 144 bp and 300 bp in BsrGI digests. To exclude fragments originating from the plastid genome, and to achieve complexity reduction, size selection (190–210 bp for HinP1I and 160–290 bp for BsrGI) was performed using 2.5% agarose gels stained with Gel Green (Biotium, Hayward, California, USA).
Size-selected fractions were treated with NEBNext Fill-in and ssDNA Isolation Module (New England Biolabs) to obtain single-stranded biotinylated fragments to use as template for PCR amplification with Illumina-compatible primers PCR 1.01 and PCR 2.01. PCR cycling conditions consisted of 98°C for 30 s followed by 20–30 cycles of 98°C for 12 s, 65°C for 30 s, 72°C for 30 s, with a final extension time of 1 min at 72°C using Phusion polymerase (New England Biolabs). Because of the very narrow range of fragment sizes that were extracted in the size selection step, 20 cycles of PCR were required for amplification of BsrGI libraries and 30 cycles were required for HinP1I libraries. The samples were purified using Agencourt AMPure XP beads (Beckman Coulter, Brea, California, USA), then quantified using the AccuBlue High Sensitivity Quantitation Kit (Biotium) on the VICTOR X3 Multilabel Plate Reader (PerkinElmer, Akron, Ohio, USA). Samples were diluted to 10 nM, then provided to the Texas A&M AgriLife Genomics & Bioinformatics Services for sequencing on the Illumina GAII and/or HiSeq 2000 instrument (Illumina, San Diego, California, USA). Table 1. Seed sources, taxonomy, and preliminary GBS statistics for a set of diploid (A 1-27, D 5-1) and allotetraploid cottons. Year Scientific name Name or designation PI no.
Origin BsrGI sorted sequences BsrGI unique stacks HinP1I sorted sequences HinP1I unique stacks. Note: PI no. = Plant Introduction number, National Plant Germplasm System. A 1-27 PI 408785 Peru 1,678,012 12,638 1,743,678 43,883 1989 G. Raimondii Ulbr. D 5-1 PI 530898 Ecuador 949,301 4215 15,323,076 8932 1984 G.
Barbadense L. K-56 PI 274514 Sinchao Chico, Piura, Peru, 3,995,793 16,668 3,440,581 17,332 2005 G. TM-1 PI 607172 College Station, Texas, USA 2,752,301 22,862 2,609,330 10,792 2002 G. Barbadense L. Pima 3-79 Sacaton, Arizona, USA 2,756,143 23,077 1,816,093 9059 2005 G.
TX-231 PI 163725 Zacapa, Zacapa, Guatemala 318,042 7895 592,566 1739 A total of ca. 54 million raw 76-bp paired-end reads were imported into the Geneious bioinformatics package to trim for quality ( P = 0.05). The length of all fragments was trimmed to 66 bp for analysis using Stacks ver. Sequences were preprocessed using the “processradtags” script, in which the 6-bp barcodes were sorted and removed, yielding 60-bp fragments. The process radtag options included: -c (clean data) and -q (discard low quality reads).
Barcode-sorted FASTQ files were processed in pairwise combinations using the “denovomap.pl” script. The de novo map parameters included: -n 3 (mismatches allowed between loci during catalog building), -m 3 (minimum number of identical, raw reads required to create a stack), -M 2 (number of mismatches allowed between loci when processing a single individual), and -t (remove, or break up, highly repetitive RAD-Tags during ustacks). Output data files were loaded to MySQL tables (Oracle Corporation, Redwood Shores, California, USA), and SNPs between taxa were annotated in a pairwise manner. We obtained an average of ca. 2 million raw sequences and ca. 15,000 unique ‘stacks’ (loci) for each sample.
To examine the partitioning of GBS markers into the gene-rich component of the genome, we used BLASTN to search the 22,862 unique BsrGI stacks and 10,792 unique HinP1I stacks from G. Hirsutum TM-1 against predicted coding DNA sequences (CDSs) and transcripts from the diploid G. Raimondii (; ) using a significance threshold of. Table 2. BLASTN results (significance value. Table 3. Numbers of BsrGI shared stacks (loci) and dual homozygous (aa/bb) marker loci across a set of intraspecific and interspecific combinations of Gossypium taxa.
Pairwise combination Shared stacks aa/bb Markers A 1/D 5 413 216 Pima 3-79/A 1 3329 1538 Pima 3-79/D 5 2123 1057 Pima 3-79/K-56 10,623 859 Pima 3-79/TM-1 12,408 2040 Pima 3-79/TX-231 5322 1183 TM-1/A 1 3172 1550 TM-1/D 5 2003 1041 TM-1/K-56 8910 2171 TM-1/TX-231 5492 575 TX-231/A 1 2031 959 TX-231/D 5 1328 690 TX-231/K-56 4836 1512 K-56/A 1 3421 1616 K-56/D 5 2072 1066. Table 4. Numbers of HinP1I shared stacks (loci) and dual homozygous (aa/bb) marker loci across a set of intraspecific and interspecific combinations of Gossypium taxa. Pairwise combination Shared stacks aa/bb Markers A 1/D 5 1201 502 Pima 3-79/A 1 2987 862 Pima 3-79/D 5 1387 351 Pima 3-79/K-56 931 62 Pima 3-79/TM-1 4921 740 Pima 3-79/TX-231 856 167 TM-1/A 1 3198 899 TM-1/D 5 1528 323 TM-1/K-56 906 182 TM-1/TX-231 961 111 TX-231/A 1 740 256 TX-231/D 5 444 119 TX-231/K-56 342 79 K-56/A 1 770 241 K-56/D 5 429 120 The proportion of aa/bb polymorphic loci to total (shared) loci was similar between BsrGI and HinP1I across all combinations of taxa. In an intraspecific comparison within G. Barbadense between cultivated variety Pima 3-79 and landrace K-56 (Peru), 6.7–8.1% of all markers showed aa/bb polymorphisms.
In an intraspecific comparison within G. Hirsutum between cultivated variety TM-1 and landrace TX-231 (Guatemala), 10.5–11.6% of markers showed aa/bb polymorphisms.
An interspecific comparison between G. Barbadense Pima 3-79 and G.
Hirsutum TM-1 showed the highest level of polymorphism (15–16.4%). These values correspond to approximate SNP frequencies of 0.0012–0.002 substitutions per base pair for intraspecific comparisons and 0.0028 substitutions per base pair for interspecific comparisons. BsrG1 and HinP1I GBS polymorphism in tetraploid Gossypium spp.
The proportion of highly informative (aa/bb) markers relative to total shared loci (stacks) in intraspecific and interspecific pairwise comparisons is shown. Barbadense cv. Pima 3-79; K-56 = G. Barbadense accession K-56; TM-1 = G. TM-1; TX-231 = G.
Hirsutum accession TX-231. To test the efficacy of selecting aa/bb markers to enrich for orthologous loci SNPs, we employed both in silico and experimental validation.
The in silico validation made use of the available A and D diploid genome sequences by determining whether the predicted aa/bb dual homozygous markers had the expected evolutionary pattern for sequences from Scenario 1. To perform this analysis, a subset of G. Hirsutum TM-1 vs. Barbadense Pima 3-79 aa/bb markers was searched against the complete genome sequences of G. Raimondii (D-genome diploid) (; ) and G. Arboreum (A-genome diploid) using BLASTN at a significance threshold of. Representative examples of the five categories of sequence alignments observed in TM-1 vs.
Pima 3-79 polymorphic markers with aa/bb marker type assignment from Stacks. Nucleotides on a black background indicate the site of the key Pima 3-79 polymorphism relative to the TM-1 reference sequence. Nucleotides on a gray background indicate additional mismatches relative to the TM-1 reference sequence. The top two lines in each category indicate the TM-1 and Pima 3-79 fragment sequences, respectively. The prefix B indicates BsrG1 markers, and H indicates HinP1I. Additional lines in the alignment represent fragments from diploid genomes along with chromosomal assignments. BGIA = Gossypium arboreum ; JGID = G.
Raimondii ; BGID = G. Raimondii ; scaf = scaffold. Table 5. Categorization of marker alignments of aa/bb markers polymorphic between Gossypium hirsutum TM-1 and G.
Barbadense Pima 3-79. Alignments included TM-1 and 3-97 alleles, along with any BLAST hits (1e-6) to sequenced A- and D-genome diploid species (;; ).
The five categories are described in the text and illustrated in. Category BsrGI HinP1I Total fragments aligned 1413 549 Category I 1183 381 Category II 24 59 Category III 30 0 Category IV 99 16 Category V 77 93 Category V without BLAST hits to diploids 7 8 Fragments assigned to a subgenome 1312 397 Fragments assigned to A T 841 234 Fragments assigned to D T 471 163 Experimental validation was performed using the PCR-based cleaved amplified polymorphic sequence (CAPS) marker method. Only a small proportion of markers were suitable for experimental validation based on the following criteria: (1) SNP had to be near the middle of the 60-bp sequence to allow for design of flanking primers, (2) flanking sequences had to have suitable G+C content for primer design (30–60%) and lack simple sequence repeats, (3) specific primers had to be designed (using the alignments) to avoid amplification of known paralogs and homeologs, and (4) the SNP had to occur within the recognition site of a commercially available restriction enzyme.
Uvlayout v2 08 keygen mac. 01 WinMacLinux crack serial keygen cd key Sep 3, 2008 PVSOL. ProMetric V8.
Geneious Crack
Only 22 TM-1 vs. Pima 3-79 markers (three HinP1I and 19 BsrGI) met all of these criteria; all of these fell into Category I (above) when examined in evolutionary alignments. Primer pairs shown in were used in PCR amplification with the KAPA3G Plant PCR kit (Kapa Biosystems, Wilmington, Massachusetts, USA), as per the manufacturer's recommended protocol. Amplification products were examined using 4% agarose gel electrophoresis (E-Gel EX, Life Technologies, Grand Island, New York, USA) before and after restriction digestion. Of the 22 CAPS markers, one marker (Bsr1616) yielded multiple PCR amplicons, none of which were of the predicted size. One marker (Bsr18072) showed unexpected partial digestion in both TM-1 and Pima 3-79 accessions. Of those markers that could be definitively scored, 20/21 (96%) showed the predicted pattern of restriction digestion for polymorphic markers that were homozygous within each of the two taxa examined.
Table 6. Cleaved amplified polymorphic sequence validation of 22 aa/bb markers that are polymorphic between Gossypium hirsutum TM-1 and G. Barbadense Pima 3-79. Locus Primer sequences (5′–3′) Enzyme Predicted cut Cut TM-1 Cut 3-79. a Predicted cut site is based on an alignment of GBS fragment sequences. b Bsr1616 yielded multiple PCR amplicons, none of which matched the expected size. 1 Robert Pyne, Josh Honig, Jennifer Vaiciunas, Adolfina Koroch, Christian Wyenandt, Stacy Bonos, James Simon, Tongming Yin, A first linkage map and downy mildew resistance QTL discovery for sweet basil (Ocimum basilicum) facilitated by double digestion restriction site associated DNA sequencing (ddRADseq), PLOS ONE, 2017, 12, 9, e0184319.
2 Caiping Cai, Guozhong Zhu, Tianzhen Zhang, Wangzhen Guo, High-density 80 K SNP array is a powerful tool for genotyping G. Hirsutum accessions and genome analysis, BMC Genomics, 2017, 18, 1. 3 Morten T. Limborg, Wesley A. Larson, Lisa W. Seeb, James E. Seeb, Screening of duplicated loci reveals hidden divergence patterns in a complex salmonid genome, Molecular Ecology, 2017, 26, 17, 4509.
4 Paul D. Blischak, Laura S. Kubatko, Andrea D. Wolfe, Accounting for genotype uncertainty in the estimation of allele frequencies in autopolyploids, Molecular Ecology Resources, 2016, 16, 3, 742.

5 Fakhriddin N. Kushanov, Alan E. Pepper, John Z. Yu, Zabardast T. Buriev, Shukhrat E. Shermatov, Sukumar Saha, Mauricio Ulloa, Johnie N. Jenkins, Abdusattor Abdukarimov, Ibrokhim Y.
Abdurakhmonov, Development, genetic mapping and QTL association of cotton PHYA, PHYB, and HY5-specific CAPS and dCAPS markers, BMC Genetics, 2016, 17, 1. 6 Ruijuan Li, John E. Erpelding, Genetic diversity analysis of Gossypium arboreum germplasm accessions using genotyping-by-sequencing, Genetica, 2016, 144, 5, 535. 7 Morten T. Limborg, Lisa W.
Seeb, James E. Seeb, Sorting duplicated loci disentangles complexities of polyploid genomes masked by genotyping by sequencing, Molecular Ecology, 2016, 25, 10, 2117. 8 Elizabeth A. Zimmer, Jun Wen, Using nuclear gene data for plant phylogenetics: Progress and prospects II. Next-gen approaches, Journal of Systematics and Evolution, 2015, 53, 5, 371.
How to Uninstall Geneious 7.1.4 Application/Software on Your Mac Unlike the software developed for Windows system, most of the applications installed in Mac OS X generally can be removed with relative ease. Geneious 7.1.4 is a third party application that provides additional functionality to OS X system and enjoys a popularity among Mac users.
However, instead of installing it by dragging its icon to the Application folder, uninstalling Geneious 7.1.4 may need you to do more than a simple drag-and-drop to the Trash. When installed, Geneious 7.1.4 creates files in several locations. Generally, its additional files, such as preference files and application support files, still remains on the hard drive after you delete Geneious 7.1.4 from the Application folder, in case that the next time you decide to reinstall it, the settings of this program still be kept. But if you are trying to uninstall Geneious 7.1.4 in full and free up your disk space, removing all its components is highly necessary.
Continue reading this article to learn about the proper methods for uninstalling Geneious 7.1.4. Manually uninstall Geneious 7.1.4 step by step: Most applications in Mac OS X are bundles that contain all, or at least most, of the files needed to run the application, that is to say, they are self-contained. Thus, different from the program uninstall method of using the control panel in Windows, Mac users can easily drag any unwanted application to the Trash and then the removal process is started.
Despite that, you should also be aware that removing an unbundled application by moving it into the Trash leave behind some of its components on your Mac. To fully get rid of Geneious 7.1.4 from your Mac, you can manually follow these steps: 1. Terminate Geneious 7.1.4 process(es) via Activity Monitor Before uninstalling Geneious 7.1.4, you’d better quit this application and end all its processes. If Geneious 7.1.4 is frozen, you can press Cmd +Opt + Esc, select Geneious 7.1.4 in the pop-up windows and click Force Quit to quit this program (this shortcut for force quit works for the application that appears but not for its hidden processes). Open Activity Monitor in the Utilities folder in Launchpad, and select All Processes on the drop-down menu at the top of the window. Select the process(es) associated with Geneious 7.1.4 in the list, click Quit Process icon in the left corner of the window, and click Quit in the pop-up dialog box (if that doesn’t work, then try Force Quit).

Delete Geneious 7.1.4 application using the Trash First of all, make sure to log into your Mac with an administrator account, or you will be asked for a password when you try to delete something. Open the Applications folder in the Finder (if it doesn’t appear in the sidebar, go to the Menu Bar, open the “Go” menu, and select Applications in the list), search for Geneious 7.1.4 application by typing its name in the search field, and then drag it to the Trash (in the dock) to begin the uninstall process. Alternatively you can also click on the Geneious 7.1.4 icon/folder and move it to the Trash by pressing Cmd + Del or choosing the File and Move to Trash commands. For the applications that are installed from the App Store, you can simply go to the Launchpad, search for the application, click and hold its icon with your mouse button (or hold down the Option key), then the icon will wiggle and show the “X” in its left upper corner. Click the “X” and click Delete in the confirmation dialog. Remove all components related to Geneious 7.1.4 in Finder Though Geneious 7.1.4 has been deleted to the Trash, its lingering files, logs, caches and other miscellaneous contents may stay on the hard disk. For complete removal of Geneious 7.1.4, you can manually detect and clean out all components associated with this application.
You can search for the relevant names using Spotlight. Those preference files of Geneious 7.1.4 can be found in the Preferences folder within your user’s library folder (/Library/Preferences) or the system-wide Library located at the root of the system volume (/Library/Preferences/), while the support files are located in '/Library/Application Support/' or '/Library/Application Support/'. Open the Finder, go to the Menu Bar, open the “Go” menu, select the entry: Go to Folder.
And then enter the path of the Application Support folder:/Library Search for any files or folders with the program’s name or developer’s name in the /Library/Preferences/, /Library/Application Support/ and /Library/Caches/ folders. Right click on those items and click Move to Trash to delete them. Meanwhile, search for the following locations to delete associated items:. /Library/Preferences/.
/Library/Application Support/. /Library/Caches/ Besides, there may be some kernel extensions or hidden files that are not obvious to find. In that case, you can do a Google search about the components for Geneious 7.1.4.
Usually kernel extensions are located in in /System/Library/Extensions and end with the extension.kext, while hidden files are mostly located in your home folder. You can use Terminal (inside Applications/Utilities) to list the contents of the directory in question and delete the offending item.
Empty the Trash to fully remove Geneious 7.1.4 If you are determined to delete Geneious 7.1.4 permanently, the last thing you need to do is emptying the Trash. To completely empty your trash can, you can right click on the Trash in the dock and choose Empty Trash, or simply choose Empty Trash under the Finder menu (Notice: you can not undo this act, so make sure that you haven’t mistakenly deleted anything before doing this act. If you change your mind, before emptying the Trash, you can right click on the items in the Trash and choose Put Back in the list). In case you cannot empty the Trash, reboot your Mac. Tips for the app with default uninstall utility: You may not notice that, there are a few of Mac applications that come with dedicated uninstallation programs. Though the method mentioned above can solve the most app uninstall problems, you can still go for its installation disk or the application folder or package to check if the app has its own uninstaller first.
If so, just run such an app and follow the prompts to uninstall properly. After that, search for related files to make sure if the app and its additional files are fully deleted from your Mac. Automatically uninstall Geneious 7.1.4 with MacRemover (recommended): No doubt that uninstalling programs in Mac system has been much simpler than in Windows system. But it still may seem a little tedious and time-consuming for those OS X beginners to manually remove Geneious 7.1.4 and totally clean out all its remnants. Why not try an easier and faster way to thoroughly remove it?
If you intend to save your time and energy in uninstalling Geneious 7.1.4, or you encounter some specific problems in deleting it to the Trash, or even you are not sure which files or folders belong to Geneious 7.1.4, you can turn to a professional third-party uninstaller to resolve troubles. Here MacRemover is recommended for you to accomplish Geneious 7.1.4 uninstall within three simple steps. MacRemover is a lite but powerful uninstaller utility that helps you thoroughly remove unwanted, corrupted or incompatible apps from your Mac.
Now let’s see how it works to complete Geneious 7.1.4 removal task. Download MacRemover and install it by dragging its icon to the application folder. Launch MacRemover in the dock or Launchpad, select Geneious 7.1.4 appearing on the interface, and click Run Analysis button to proceed. Review Geneious 7.1.4 files or folders, click Complete Uninstall button and then click Yes in the pup-up dialog box to confirm Geneious 7.1.4 removal. The whole uninstall process may takes even less than one minute to finish, and then all items associated with Geneious 7.1.4 has been successfully removed from your Mac! Benefits of using MacRemover: MacRemover has a friendly and simply interface and even the first-time users can easily operate any unwanted program uninstallation.
With its unique Smart Analytic System, MacRemover is capable of quickly locating every associated components of Geneious 7.1.4 and safely deleting them within a few clicks. Thoroughly uninstalling Geneious 7.1.4 from your mac with MacRemover becomes incredibly straightforward and speedy, right? You don’t need to check the Library or manually remove its additional files. Actually, all you need to do is a select-and-delete move. As MacRemover comes in handy to all those who want to get rid of any unwanted programs without any hassle, you’re welcome to download it and enjoy the excellent user experience right now! This article provides you two methods (both manually and automatically) to properly and quickly uninstall Geneious 7.1.4, and either of them works for most of the apps on your Mac.
If you confront any difficulty in uninstalling any unwanted application/software, don’t hesitate to apply this automatic tool and resolve your troubles.
Comments are closed.